Articular cartilage – structure, biomechanics and damage aetiology
1) Articular cartilage – basic information
a. Morphology of articular cartilage
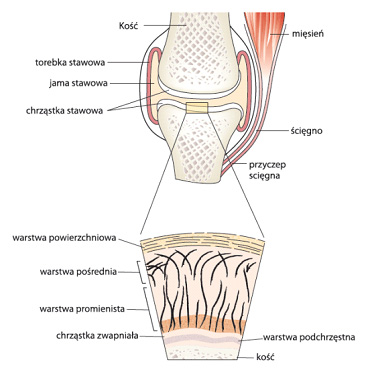
Figure 1. Schematic structure of the joint and cross-sectional layers of articular cartilage
The most important characteristic of articular cartilage, determining its primary function, is its high resistance to abrasion. Therefore, it is located around the articular ends of bones, preventing them from wearing off as a result of the locomotor system’s work. High elasticity of the tissue allows it to change shape under the influence of forces transmitted during the body movement, bearing to a large extent the load acting on the joint. Articular surfaces of the human body are composed of two types of this supporting variety of connective tissue: the vitreous (majority) and fibrous. With age, the cartilage changes its colour taking on more yellow, and its elasticity and thickness change as well. This results in reduced cushioning of joints and their increased susceptibility to injury.
In a typical joint there are two surfaces acting on each other: concave – the acetabulum – and convex – the head (usually has a more rigid cartilage). With age, the cartilage thickens in the centre of the head, while within the centre of acetabulum it is getting thinner. Cartilage thickness varies depending on the joint type and the location in it. The thickest one has 6 mm and is found on the fibular surface of the patella, while the thinnest has about 0.2 mm. The average cartilage thickness is in the range of 0.5-2 mm. It seems quite surprising that there has been observed no correlation between the volume of joint cartilage, and the age, weight or body height.
Articular cartilage is devoid of blood or lymphatic vessels, and is not innervated. Nutrition of this tissue occurs almost entirely by diffusion (free transfer) of the substance from the articular cavity, and, in a small part (1-7%) through the capillary vessels that depart from the subchondral layer to the calcified layer of the cartilage. Lack of perichondrium practically prevents the regeneration of articular cartilage, which does not mean that this process is impossible.
b. The structure of articular cartilage
Articular cartilage has a layered structure (see Figure 1). Its most external part of the surface layer, in the immediate vicinity of the mouth of the articular cavity, is devoid of cells but contains numerous, parallel to the articular surface, collagen fibres (type II, IX and XI), small amount of glycosaminoglycans (GAGs) and is rich in hyaluronic acid. The surface layer, accounting for approx. 10% of the cartilage thickness, acts as protective membrane for the lower lying cartilage structures. In the next layer, constituting 40% of the cartilage volume, collagen fibres are arranged obliquely. The two deepest layers – 50% of the cartilage thickness, are the stratum radiatum in which the collagen fibres run perpendicular, and the calcified cartilage layer directly in contact with the subchondral bone surface. This complex, layered arrangement of collagen fibres ensures for the articular cartilage the proper strength and effective dissipation of forces acting on the joint during the body movement, providing effective cushioning and protecting the locomotor system from damage.
Figure. 2. Hypothetical schematic structure of cartilage “aggrecan”
Chondrocytes (cartilage cells) represent only about 1% of the volume of cartilage and are responsible for the synthesis, catabolism and general homeostasis of the cartilage. They remain suspended in the matrix which consists mainly of water (60% -80% of the total weight), collagen (60% dry weight) and proteoglycans (30% dry weight). The physiology of cartilage and its characteristic visco-elastic properties stem directly from the fact that water is its main ingredient.
c. Synovial fluid and protection of cartilage functionality
Articular cavity is filled with a viscous fluid composed of hyaluronic acid, lubricin, proteinases and collagenases. It is secreted by cells of the synovial membrane, and forms on its surface a layer of about 50 microns, while also “sipping” into microfolds and hollows present on the articular surface, filling in all the empty spaces. The most important function of synovial fluid is the reduction of friction between the surfaces of articular cartilage. The polysaccharide structure of hyaluronic acid ensures, owing to the sugar-sugar and/or sugar-protein interactions, the formation of a sticky barrier on the surface of cartilage, effectively preventing its degradation by minimising the release of proteoglycans from extracellular matrix. The effect of a significant reduction of friction within the joint has been achieved, in animal studies, also with oral administration of chondroitin sulfate, which has directly translated into increased viscosity of synovial fluid – the state attained through the physiological presence hyaluronic acid in the articular cavity.
2) Biochemical structure of chondroitin sulfate (SC)
Figure 3. Chemical structure of the chondroitin sulfate chain monomer
The polysaccharide, which chondroitin sulfate (SC) is, constitutes an essential component of the extracellular matrix of all tissues. Its main function is the building of proteoglycans by creating a covalent bond with proteins. The basic “building block” (monomer) of the cartilage polysaccharide, i.e. chondroitin sulfate, is a disaccharide consisting of a molecule of D-glucuronic acid linked by the b 1 → 3 bond with a molecule of N-acetyl-D-galaktosamine (see Figure 3). These monomers are linked by the b 1 → 4 bonds. Polysaccharide chains of GAGs, after the synthesis, are modified by the addition to the galactosamine molecules of sulfonic groups at position 4 or 6. Ionised sulfo groups, together with carboxyl groups, give the GAG chain a negative charge, the importance of which is described later in the text. It appears that the distribution pattern of sulfo groups in chondroitin monomers is different in the human articular cartilage depending on the age and the cartilage surface. In the growing cartilage, SC chains are composed of 30-40 monomers with an equal ratio of carbon sulfonation in positions 4 and 6, while in the mature tissue chondroitin sulfate chains consist of ca. 20 monomers, and sulfonation is predominantly in position 6.
3) Biomechanics of articular cartilage
Articular surface only appears to be smooth, but in fact it has a very complex structure, which consists of a number of folds, minor depressions and even lower level ridges. With such a structure, the surface of articular cartilage can achieve very low friction coefficient, ranging from 0.01 to 0.02 (for the knee, it is only 0.002). This value is inversely proportional to the increase in the load and is also significantly reduced by covering the surface of the joint with synovial fluid.
The biomechanical properties of articular cartilage stem mainly from the physicochemical properties of glycosaminoglycans, which account for 80-90% of proteoglycans. The presence of hydroxyl, ionized sulfonic and carboxyl groups arranged in series results in proteoglycans attracting a large number of water molecules owing to electrostatic forces. Water dipoles, due to the accumulation of negative charges, arrange in multi-layer structures that surround the charges attracting them. This leads to the creation of osmotic gradients and induction of the internal swelling pressure. To put it more descriptively: articular cartilage can be visualised as a mattress filled with water, in which the liquid pressure is maintained through the continuous operation of a water pump. The surface of the mattress is the outer part of the surface layer of articular cartilage, and proteoglycans binding water act as the pump. When articular cartilage is subjected to load, the electrostatically bound water is squeezed out from between the structures of proteoglycans. In consequence, negatively charged groups come closer to each other and cause a further increase in pressure, additionally hardening the cartilage. When the pressure passes, water dipoles return to the vicinity of negative charges, restoring the ordered structure of cartilage. The tensions in the cartilage are not uniform. During the load, the deeper layers are subject to compression, while the more surface ones – to stretching (hence the latter are defined as having greater rigidity). It has been shown that cartilage responds to a reduction of its volume as a result of the load acting on it. This has been evidenced by studies conducted on volunteers in whom, after a series of 50 deep knee bends, the patellar cartilage volume was measured, and after 3-7 minutes it was reduced by 6%, while after 8-12 min – by 5%, in comparison with the baseline status at rest. With the passage of time, after the stimulus is removed, the size of articular cartilage returns to the initial parameters. This is owing to a progressive restoration of cartilage structure stemming from the return of water dipoles to the vicinity of negative charges and a full restoration of the hydrodynamic properties of articular cartilage.
4) Aetiology of articular cartilage damage.
The contact area around the joint is far from ideal, since the area of exposure is often very small. As a result, the load acting on the joint spreads over a very small area, which may act damaging on this site and lead to stunted growth in the area of cartilage. The adaptive response of the body to the prevailing unfavourable conditions is to accelerate the growth of articular cartilage in less exposed sections, in order to increase the surface of impact and thereby spread the force over a larger area. Owing to this, a minimisation of the damaging effect is made possible. One of the most frequently suggested mechanisms of the development of degenerative condition within the articular cartilage states that the main reason for this is the high stimulus dynamics – high force and, importantly, short acting. Its damaging effects stem from the fact that articular cartilage and the layers beneath it need time to adapt their shape to the rapidly changing mechanical conditions. With too strong a stimulus, this mechanism cannot work (required is a longer duration of static load) and the intricate structure, based on the concept of a mattress filled with water, may become damaged and lose its hydrodynamic properties. This is how the processes of proteoglycan depolymerisation and articular cartilage degradation potentially start.
It has been found that normal, physiological strain on joints is necessary to maintain a balance between the synthesis and degradation of extracellular matrix components, as well as to retain the rate of cartilage structure remodelling, necessary for the proper biomechanics of articular cartilage. On the other hand, it is also true that excessive mechanical load on articular cartilage structures, associated with obesity for instance, is a key aetiological factor for the development and progression of arthritis. It is believed that the disturbance of articular cartilage biomechanics results from changes in the chondrocyte shape and, consequently, their altered interaction with the extracellular matrix, which in turn may activate different intracellular signalling pathways, affecting the secretion of compounds produced in chondrocytes. To present it in more detail: excessive mechanical pressure on the articular cartilage can alter the physicochemical properties of the matrix (pH, ionic strength, etc.), and consequently the ease of diffusion and levels of growth factors and cytokines, and thus alter the characteristics of the impact of ligand-receptor, which in turn can give very abnormal characteristics of intracellular signalling pathways.
5) Anabolic-catabolic balance within the articular cartilage
a. Proteoglycan biosynthesis and its regulation
In articular cartilage tissue, over 50% of chondrocytes exhibit signs of entering the programmed death (apoptosis) pathway, while in a typical cartilage, only 10% of cells do so. This reflects just how dynamic this site of our body is, and how efficiently the processes of decomposition and regeneration of tissue structures must take place within the joint – and what is more, how precisely they need to be regulated.
The synthesis of proteoglycans requires proteins, activated carbohydrates (most of them are derivatives of uridine diphosphate – UDP) and enzymes (glucosyltransferases and sulfotransferases). The core proteins of proteoglycan aggregates are synthesized in ribosomes in the rough endoplasmic reticulum and secreted into its lumen. Then, in the Golgi apparatus, polysaccharide chains of chondroitin and keratan are attached to them. The last step is the sulfonation of specific carbon atoms. The process of synthesis of a single proteoglycan aggregate requires more than 10,000 enzymatic reactions.
The cartilage proteoglycan synthesis is inhibited by uridine diphosphate N-acetylglucosamine (UDP GlcNac), an aminotransferase inhibitor activated by uridine diphosphate xylose – an initiator of polysaccharide chain synthesis. In addition, each newly synthesised monomer (disaccharide) of glycosaminoglycan chain activates further elongation of the forming structure.
b. Mechanism of articular cartilage degradation
In natural conditions, chondroitin sulfate within the extracellular matrix of the cartilage is broken down as a result of activity of liposomal enzymes secreted by the connective tissue cells: glycosidases (N-acetylglucosaminidase, galactosidase, glucuronidase) and chondroitinases that destroy the bonds between N-acetyl-D-glucosamine and D-glucuronic acid, leading to breakdown in the monomers forming the long chain of glycosaminoglycan. This mechanism constitutes the natural process of modification of tissue structures, also used in the removal of its worn out and/or damaged fragments.
However, the degradation process itself can “escape” the body’s control and occur on a much larger scale, affecting the other components of cartilage matrix. This takes place during the development of inflammation in the cartilage. The process involves white blood cells (leukocytes) that by releasing enzymes – elastases – disorganize (degrade) a complicated structure of proteoglycan and collagen aggregates. In this “massive” degradation of articular cartilage there are often involved chondrocytes, secreting to the extracellular matrix such enzymes as cathepsin B, metalloproteinases, and serine proteases, which extremely efficiently contribute to the further destruction of proteoglycan and collagen, to effectively deprive the cartilage of its characteristic visco-elastic properties.
Fortunately, it appears that this process can be effectively countered. As already mentioned, the administration of chondroitin sulfate (SC) resulted in an improvement in the viscosity of synovial fluid, reduction in the friction between articular surfaces and thereby decreased pain associated with arthritis. It has been evidenced that SC is able to inhibit the destructive activity of enzymes mentioned above through the creation of electrostatic bonds between the negatively charged sulfate groups of GAGs and the positively charged active sites of proteolytic enzymes, thus blocking the latter. The strength of the protective effect of SC on the articular cartilage is the greater, the higher the molar mass of glycosaminoglycan chains in the tissue milieu. Of importance here is the pattern of sulfonation of N-acetyl-D-galactosamine molecules building the chondroitin sulfate monomers. It appears that much more effective inhibition of the inflammatory process occurs when isomers sulfonated in position 6 predominate in the compound.
6) Summary
Nature often comes to our aid in the treatment of various diseases. This is also the case in impaired functioning within the musculoskeletal system. Chondroitin sulfate is but one of several substances providing effective relief and contributing to the improvement in the condition of our joints. Its effectiveness, as well as that of several other chemicals, is increasingly more often evidenced by results of clinical trials. Awareness of what is happening in our body gives us knowledge about how to counteract the adverse changes occurring in it. I hope that with this article I was able to arouse your curiosity about the structure, functioning and balance of biochemical processes occurring in the articular cartilage. Meanwhile, in terms of “weapons” to fight the deteriorating health of our joints and, above all, prevention of these adverse changes, the subsequent articles in this series will address these issues. Wishing all the Perfect Body readers good health, I encourage you to reach for the next issues of our magazine.
Piotr Kaczka, PhD Eng
Bibliografia:
1) Bali JP, Cousse H, Neuzil E. Semin. Biochemical basis of the pharmacologic action of chondroitin sulfates on the osteoarticular system. Arthritis Rheum. 2001 Aug;31(1):58-68.
2) Sauerland K, Plaas AH, Raiss RX, Steinmeyer J. The sulfation pattern of chondroitin sulfate from articular cartilage explants in response to mechanical loading. Biochim Biophys Acta. 2003 Jul 30;1638(3):241-8.
3) Ciszek B. Morfologia i funkcja chrząstki stawowej. Acta Clinica 2001 1:10-14
4) Buckwalter JA, Mankin HG: Articular cartilage I Tissue design and chondrocyte matrix interactions J. Bone Joit Surg (Am) 1977 79:600 – 611
5) Eckstein F, Winzheimer M, Westhoff J, Schnier M, Haubner M, Englmeier KH, Reiser M, Putz R: Quantitative relationships of normal cartilage volumes of the humen knee joint — assessment by magnetic resonance imaging. Anat Embryol. 197:383 – 390 1998.
6) Eckstein F, Tieschky M, Faber SC, Haubner M, Kolem H, Englmeier K-H Reiser M: Effect of physical exercise on cartilage volume and thicness in vivo: MR imaging study. Radiology 207:243 – 248 1998
7) Frost HM: Joint anatomy, design, and arthroses: insights of the utah paradigm. Anat. Rec. 255:162 – 173 1999
8) Modl JM, Sether LA, Haughton VM, Kneeland JB: Articular cartilage: correlation of histologic zones with signal intensity at MR imaging Radiology 1991 181:853 – 855.
9) Buckwalter JA, Mankin HJ: Articular cartilage: degeneration and osteoarthritis, repair, regeneration, and transplantation, AAOS Instr. Course Lect. 47 (1998) 487–504.
10) Bayliss MT, Osborne D, Woodhouse S, Davidson C: Sulfation of chondroitin sulphate in human articular cartilage. The effect of age, topographical position, and zone of cartilage on tissue composition. J Biol Chem 1999;274:15892-900.
11) Caplan AJ: Cartilage. Sci Amer 1984;251:82-90.
12) Neuzil E, Cassaigne A: L’eau, une mole´cule tre´s particulie`re. L’alimentation et la vie. 1992;92:5-18.
13) Hata RI, Nagai Y: A low-sulfated chondroitin sulphate in rat blood: an acidic glycosaminoglycan with a high metabolic rate. Biochim Biophys Acta 1978;543:149-55.
14) Conte A, Volpi N, Palmieri L, Bahous I, Ronca G: Biochemical and pharmacokinetic aspects of oral treatment with chondroitin sulphate. Arzneim Forsch/Drug Res 1995;45:918-25.
15) Baici A, Bradamante P: Interaction between human leukocyte elastase and chondroitin sulphate. Chem Biol Interac 1984;51:1-11.
16) Nishikawa H, Mori I, Umemoto J: Influences of sulfated glycosaminoglycans on biosynthesis of hyaluronic acid in rabbit knee synovial membranes. Arch Biochem Biophys 1985; 240:145-53.
BACK ›